中国海洋湖沼学会主办。
文章信息
- 石仞, 张蓬, 曹胜凯, 邢戎光, 纪浩, 葛林科, 张翠荣. 2022.
- SHI Ren, ZHANG Peng, CAO Sheng-Kai, XING Rong-Guang, JI Hao, GE Lin-Ke, ZHANG Cui-Rong. 2022.
- 高效液相色谱-串联质谱法同时测定海洋沉积物中27种有机磷酸酯
- SIMULTANEOUS DETERMINATION OF 27 ORGANOPHOSPHATE ESTERS IN MARINE SEDIMENTS IN HIGH-PERFORMANCE LIQUID CHROMATOGRAPHY-TANDEM MASS SPECTROMETRY
- 海洋与湖沼, 53(6): 1370-1384
- Oceanologia et Limnologia Sinica, 53(6): 1370-1384.
- http://dx.doi.org/10.11693/hyhz20220200030
文章历史
-
收稿日期:2022-02-08
收修改稿日期:2022-04-07
2. 咸阳市生态环境环境局 陕西咸阳 712099
2. Xianyang Ecology and Environment Bureau, Xianyang 712099, China
由于溴代阻燃剂, 尤其是多溴联苯醚已被全球逐步禁用, 作为其主要替代品的有机磷阻燃剂(OPFRs)生产和使用水平大大提高, 其中有机磷酸酯(organophosphate esters, OPEs)是使用最广泛的OPFRs (Van der Veen et al, 2012; 耿存珍等, 2016)。目前OPEs被应用于塑料、纺织、电子机械、建筑家居等多个领域, 多用作阻燃剂或增塑剂, 混凝土、漆、液压油以及地板抛光剂中的抗磨剂和消泡剂, 也在湿法冶金中起到非离子萃取剂的作用(Peverly et al, 2015; Xing et al, 2018; Zou et al, 2018)。OPEs与聚合物产品并非以化学键结合, 溶解、挥发或人为磨损破坏等物理因素, 都可能使其脱离材料进入环境, 进而通过地表径流、水汽交换和大气干湿沉降远距离输送(Ma et al, 2017; Ren et al, 2019; Zeng et al, 2020)。OPEs已普遍存在于空气(郭志明等, 2016; Rauert et al, 2018; Na et al, 2020)、土壤及植物(印红玲等, 2016; Campo et al, 2017; 邓旭等, 2019)、淡水和海洋水体(Bollmann et al, 2012; Kim et al, 2018; Xing et al, 2018; 吴迪等, 2019)及沉积物(Ma et al, 2017; Wang et al, 2017; Zhong et al, 2018)等各种环境介质中。由于OPEs具有环境持久性且大多数OPEs是中至高疏水的亲脂性化合物, 输入到海水中后倾向于与悬浮颗粒物结合进而在沉积物中长期积累, 很难得到转化和降解, 因而沉积物是OPEs的重要“汇”(Wang et al, 2017, 2019)。一些研究报道了国内外典型海洋沉积物中OPEs的赋存状况, 地中海西北部表层沉积物中检出的九种OPEs的浓度和(Σ9OPEs)范围为13~49 ng/g (Schmidt et al, 2021); 我国渤海和黄海表层沉积物中检出的Σ7OPEs浓度范围为8.12~98.00 ng/g(Zhong et al, 2017); 韩国沿海及附近港口的表层沉积物中检出的Σ13OPEs浓度范围为2.18~347 ng/g (Choi et al, 2020), 海洋表层沉积物中OPEs的残留在几十至几百ng/g之间。
底栖生物摄食沉积物颗粒, 吸附在沉积物中的OPEs会通过食物网产生的生物富集效应和生物放大效应, 进入高营养级猎食者和人类体内(Wang et al, 2019)。已在浮游动植物、双壳类动物和无脊椎动物等海洋生物体内检测到OEPs的存在, 浓度较低在几至几百ng/g之间, 甚至在更高营养级的鱼类和海鸥等体内以及人类母乳中也有OPEs残留, 浓度高达几十至几千ng/g (Sundkvist et al, 2010; Wei et al, 2015; Pantelaki et al, 2020)。有毒理学研究发现, OPEs具有多种毒性效应, 会对生物和人类健康产生潜在的危害, 如三(2-氯异丙基)磷酸盐(TCIPP)和三(2-羧乙基)膦(TCEP)对人体产生致癌性; 三聚磷酸酯(TPHP)和三丁基磷酸盐(TNBP)具有神经毒性, 接触致敏效应, 还会降低生育能力; 三(2-丁氧基乙基)磷酸酯(TBEP)可降低红细胞胆碱酯酶活性, 而磷酸三甲酯(TMP)和磷酸三乙酯(TEP)则通过激活核受体破坏甲状腺内分泌系统(Li et al, 2014; Been et al, 2018; Xing et al, 2018; Zeng et al, 2020)。在海洋沉积物中往往存在多种混合污染物的共同作用, 然而大多数实验室毒理学研究都集中在个别物质对生物的毒性作用, 对此类联合毒性效应产生的健康风险还关注甚少(Cristale et al, 2013)。显然, 要评估沉积物等复杂环境基质对生物和人类产生的潜在健康风险, 获得其中OPEs的含量水平是首要前提, 而建立关于海洋沉积物样品的分析方法是至关重要的一步。
目前, 气相色谱-质谱联用(GC-MS)是环境中OPEs检测常用的方法, 尽管气相色谱(GC)具有良好的分离能力, 但对复杂环境样品的分析存在选择识别能力有限和稳定性低等缺陷(Björklund et al, 2004; Quintana et al, 2008)。有研究发现, 聚磷酸酯等分子量大的OPEs不适用于GC测定, 一些OPEs还会出现拖尾峰, 如三苯基氧膦(TPPO)和三(2-丁氧基乙基)磷酸酯(TBEP)(Rodil et al, 2005; Teo et al, 2015)。二酯和单酯类OPEs的疏水性、非挥发性和强酸性(解离常数pKa≤1)比三酯要小得多, 在环境中以二价阴离子或单阴离子的离解状态存在且更具极性, GC-MS主要用于非极性物质的检测, 二酯和单酯类OPEs则需要通过衍生化处理后才能测定(Quintana et al, 2008; Reemtsma et al, 2008; Suo et al, 2018)。基于四极杆的GC-MS选择离子检测(SIM)和基于离子阱的气相色谱-串联质谱(GC-MS/MS)检测灵敏度低, 且GC检测常用的电子轰击离子源(EI源)和化学电离离子源(CI源)难以产生分子离子(Quintana et al, 2008)。高效液相色谱-串联质谱(HPLC-MS/MS)采用多反应监测(MRM)-三重四级质谱(QqQ-MS)检测, 具有较高的选择性、抗干扰能力和灵敏度, 且电喷雾离子源(ESI源)能够产生分子离子, 也可以产生多电荷离子, 因而对于分子量大的化合物也可分析, 适用于复杂基质样品中OPEs的微量分析(Wu et al, 2017; Wang et al, 2020; 闫振飞等, 2020)。目前研究中使用HPLC- MS/MS测定复杂基质中OPEs的方法多集中于对环境中常检出的较少种类化合物的分析, 因而建立可以同时测定多种OPEs的高效、简便、高灵敏度和低检出限的分析测定方法十分必要。本文对HPLC-MS/MS的色谱和质谱参数以及前处理方法进行了优化, 建立了同时测定海洋沉积物中27种OPEs的高效分析方法。
1 材料与方法 1.1 仪器与试剂材料本实验所采用的仪器设备有: Agilent 1260 Infinity II高效液相色谱和Agilent 6470 LC/TQ三重四级杆质谱仪(美国Agilent公司), BSA224S(Max: 220 g, d=0.1 mg)电子天平(赛多利斯科学仪器(北京)有限公司), KQ-500DB型数控超声波清洗器(昆山市超声仪器有限公司), TDZ4-WS台式低速离心机(湖南赫西仪器装备有限公司), RE-2000B型旋转蒸发仪(上海亚荣生化仪器厂), NAI-DCY-12Y圆形水浴氮吹仪(上海那艾精密仪器有限公司)。
固相萃取小柱(solid phase extraction, SPE): Oasis®HLB 6 cc/500 mg (美国Waters公司)、CNWBOND LC-C18 (容积6 mL/500 mg)和CNWBOND Si硅胶SPE (6 mL/500 mg)(上海安谱实验科技股份有限公司)。3种同位素替代标: 氘代磷酸三苯酯(TPP-d15, 纯度为98%), 氘代三(2-氯乙基)磷酸酯(TCEP-d12, 纯度为98%)和氘代磷酸三丁酯(TBP-d27, 纯度为98%~99%)均购自剑桥同位素实验室(美国); 有机溶剂: 乙腈(ACN)和甲醇(MeOH) (HPLC级, 美国Thermo Fisher Scientific公司), 乙酸乙酯(EA)和二氯甲烷(DCM)(色谱纯, 美国Tedia公司); 实验用水为自制Milli-Q超纯水(18.24MΩ·cm)。
1.2 仪器条件采用Zorbax Eclipse Plus C18色谱柱(2.1 mm× 150 mm, 3.5-Micron, Agilent), 柱温25 ℃, 进样量1 μL, 流速0.35 mL/min。采用带有安捷伦喷射流技术的大气压电喷雾离子源(ESI with Agilent Jet Steam Technology, ESI-AJS), 在多反应监测(MRM)的正离子模式下测定, 毛细管(Capillary)电压为4 000 V(正离子模式), 喷嘴电压(Nozzle Voltage)为500 V, 干燥气(Drying Gas)流速为5 L/min, 温度为350 ℃, 雾化气(Nebulizing Gas)压力为45 psi, 鞘气(Sheath Gas)流速为11 L/min, 温度为250 ℃。流动相梯度洗脱程序和质谱参数如表 1和表 2所示。
时间/min | 0.002 5%的甲酸水溶液比例/% | 甲醇比例/% |
0 | 45 | 55 |
0.5 | 20 | 80 |
10 | 0 | 100 |
15 | 0 | 100 |
16 | 45 | 55 |
25 | 45 | 55 |
化合物 | 分子式 | 保留时间 /min |
母离子 | 子离子 | 毛细管碎裂电压 /V |
碰撞能量 /eV |
磷酸三甲酯(TMP) | C3H9O4P | 1.207 | 141.02 | 109.1 | 111 | 17 |
79.3 | 25 | |||||
磷酸三乙酯(TEP) | C6H15O4P | 2.318 | 183.00 | 99.2 | 61 | 21 |
81.3 | 53 | |||||
三(2-羧乙基)膦(TCEP) | C6H12Cl3O4P | 3.318 | 284.90 | 98.8 | 100 | 25 |
63.0 | 25 | |||||
磷酸三丙酯(TPrP) | C9H21O4P | 4.662 | 225.12 | 99.2 | 81 | 21 |
141.1 | 5 | |||||
磷酸三异丙酯(TiPP) | C9H21O4P | 4.960 | 225.12 | 99.0 | 86 | 17 |
81.0 | 57 | |||||
四氯(2-氯乙基)二氯异戊二磷酸(V6) | C13H24Cl6O8P2 | 5.123 | 582.70 | 234.9 | 200 | 41 |
65.0 | 60 | |||||
磷酸三苯酯(TPP) | C18H15O4P | 6.063 | 327.07 | 214.9 | 136 | 29 |
152.1 | 45 | |||||
磷酸三氯丙基酯(TCPP) | C9H18O4PCl3 | 6.064 | 327.00 | 99.1 | 136 | 49 |
磷酸三(1-氯化异丙基)酯 (1, 2-)TCPP |
C9H18O4PCl3 | 6.064 | 327.00 | 98.9 | 136 | 41 |
77.1 | 49 | |||||
磷酸三(2-氯丙基)酯(2-TCPP) | C9H18O4PCl3 | 6.064 | 327.00 | 99.0 | 136 | 41 |
77.1 | 45 | |||||
二(2, 3-二溴丙基)磷酸酯 (B(2, 3-d)P) |
C6H11O4PBr4 | 6.462 | 498.70 | 298.8 | 170 | 6 |
98.9 | 17 | |||||
磷酸甲苯二酯(CDP) | C19H17O4P | 6.638 | 341.00 | 151.9 | 175 | 49 |
91.0 | 45 | |||||
磷酸三丁酯(TBP) | C12H27O4P | 6.795 | 267.16 | 99.2 | 111 | 21 |
155.1 | 9 | |||||
磷酸二乙酯(mono & di) | C4H11O4P | 6.796 | 155.04 | 98.9 | 220 | 9 |
58.0 | 60 | |||||
磷酸-2-丁氧代乙醇酯 (TBEP) |
C18H39O7P | 7.292 | 399.24 | 299.0 | 126 | 13 |
199.0 | 13 | |||||
磷酸-1, 3-亚苯基四苯酯(RDP) | C30H24O8P2 | 7.554 | 574.90 | 418.9 | 200 | 41 |
480.9 | 41 | |||||
三邻甲苯基磷酸酯(TOCP) | C21H21O4P | 8.147 | 369.12 | 91.0 | 195 | 45 |
165.0 | 57 | |||||
三对甲苯基磷酸酯(TPCP) | C21H21O4P | 8.147 | 369.12 | 91.0 | 200 | 49 |
165.0 | 60 | |||||
磷酸三间甲苯酯(TMTP) | C21H21O4P | 8.147 | 369.12 | 91.0 | 200 | 45 |
165.0 | 60 | |||||
磷酸二苯异辛酯(EHDPP) | C20H27O4P | 8.782 | 363.16 | 251.0 | 106 | 9 |
152.1 | 53 | |||||
磷酸三正戊酯(TPeP) | C15H33O4P | 9.092 | 309.21 | 99.0 | 121 | 25 |
81.0 | 60 | |||||
双酚A双(磷酸二苯酯)(BADP) | C39H34O8P2 | 9.989 | 693.00 | 336.8 | 200 | 37 |
336.9 | 37 | |||||
三(2, 3-二溴丙基)磷酸酯 (TDBPP) |
C9H15O4PBr6 | 9.989 | 692.58 | 367.1 | 265 | 41 |
三(2-异丙基苯)磷酸酯(TiPPP) | C27H33O4P | 10.872 | 453.21 | 327.0 | 190 | 33 |
411.0 | 17 | |||||
三(三溴新戊基)磷酸酯 (TTBNP) |
C15H24O4PBr9 | 10.894 | 1018.40 | 144.9 | 180 | 44 |
105.2 | 92 | |||||
磷酸异癸酯(DPDP) | C22H31O4P | 12.452 | 391.10 | 251.0 | 290 | 9 |
56.9 | 41 | |||||
磷酸三辛酯(TEHP) | C24H51O4P | 14.080 | 435.35 | 99.1 | 106 | 13 |
113.2 | 9 | |||||
TPP-d15 | C18D15O4P | 5.985 | 342.16 | 82.1 | 180 | 53 |
81.5 | 60 | |||||
TCEP-d12 | C6D12Cl3O4P | 6.113 | 297.03 | 281.0 | 131 | 13 |
TBP-d27 | C12D27O4P | 6.632 | 294.33 | 102.0 | 121 | 102 |
83.0 | 83 | |||||
注: 两个子离子, 第一个是定量离子, 第二个是定性离子 |
称取5 g冷冻干燥的沉积物样品于离心管, 加入TPP-d15、TCEP-d12和TBP-d27三种替代标各20 ng。用30 mL乙酸乙酯(EA)和乙腈(ACN)混合溶剂(EA/ACN体积比3︰7)超声萃取30 min, 在低速离心机3 000 r/min的转速下离心10 min, 循环2次, 收集两次所得上清液于鸡心瓶中。将提取液经水浴旋蒸浓缩至2 mL, 加超纯水定容至10 mL, 再用5 mL EA过LC-C18固相萃取(SPE)小柱洗脱2次, 然后氮吹至近干, 用MeOH溶液经0.22 μm滤膜过滤后定容至0.5 mL。
LC-C18固相萃取步骤: 用5 mL EA预处理净化固定相, 泵抽3 min至干, 然后用5 mL MeOH和5 mL超纯水活化小柱(约4 mL/min的流速, 不抽干); 上样溶剂采用20%的ACN水溶液10 mL, 保持流速5~8 mL/min, 上样结束后用10 mL 10%的MeOH水溶液淋洗去除多余杂质, 抽3 min至干, 最后用5 mL EA洗脱2次(流速1 mL/min), 并收集两次所得洗脱液。
2 结果与讨论 2.1 色谱条件优化MeOH和ACN为常用的两种液相流动相(Martínez-Carballo et al, 2007; Luo et al, 2014; 刘娴静等, 2021)。比较水-MeOH和水-ACN两种流动相体系对27种OPEs的洗脱能力, 如图 1所示。虽然一些OPEs在水-ACN体系中的响应强度高于水-MeOH体系, 但水-MeOH体系中更多种类的OPEs得到洗脱分离。在水-ACN体系中磷酸三氯丙基酯(TCPP)同分异构体[包括TCPP、磷酸三(1-氯化异丙基)酯[(1, 2-)TCPP]和磷酸三(2-氯丙基)酯(2-TCPP)]、磷酸甲苯二酯(CDP)和磷酸三辛酯(TEHP)没有得到洗脱分离, 而在水-MeOH体系中除四氯(2-氯乙基)二氯异戊二磷酸(V6)以外, 其他化合物都实现了较好的洗脱分离。且有研究证明, ESI源在正模式下能有效地产生三酯类OPEs的质子化分子离子, 而随后的碰撞诱导解离(CID)产生碎片离子则依赖于醇的酯化作用, 同样二烷基酯类OPEs阴离子的CID也会依赖于醇(Quintana et al, 2008; Reemtsma et al, 2008)。经比较选择水-MeOH体系作为两种流动相更为合适。
![]() |
图 1 流动相种类对有机磷酸酯OPEs响应强度的影响 Fig. 1 Influence of mobile phase type on response strength of organophosphate esters (OPEs) |
在ESI-AJS源正离子模式下, 流动相中通常加入挥发性酸作为有机改性剂, 一般选择甲酸, 为目标分析物提供必要的质子源, 以提高电离效率(Rodil et al, 2005; Luo et al, 2014)。流动相中的酸性程度影响化合物生成[M+H]+加和离子的效率, 进而影响方法的灵敏度(王九明等, 2021)。因此对水流动相中的甲酸添加量进行优化, 比较不同体积分数甲酸的作用效果, 如图 2所示。现有研究中广泛应用含0.1%的甲酸作为改性剂, 但其响应效果并不理想。各OPEs在含0.02%甲酸水流动相中的响应强度略高于含0.01%和0.03%甲酸水流动相中的响应强度, Xing等(2018)和Chen等(2019)在液相分析中用到0.02%甲酸水溶液作为水流动相。
![]() |
图 2 流动相甲酸添加量对OPEs响应强度的影响 Fig. 2 Influence of formic acid volume fraction in mobile phase on ions abundance of organophosphate esters (OPEs) |
当甲酸浓度为0.002 5%~0.005%体积分数之间时, 各OPEs单体的响应强度最高, 且此范围内的响应强度值远大于甲酸含量为0.02%时的响应强度, 其中多于1/2的OPEs单体在甲酸含量为0.002 5%的水流动相中的响应强度信号更高。表明OPEs在pH值为3.3~4.6的流动相水溶液中电离程度最高, 最终确定在水-MeOH流动相体系中, 以甲酸含量为0.002 5%的水溶液作为水流动相, 在此pH条件下为目标化合物提供了更好的缓冲能量, 也会产生更稳定的分离和电离效果(Rodil et al, 2005)。
2.2 样品前处理条件优化 2.2.1 超声萃取溶剂优化由于27种OPEs的辛醇-水分配系数(logKow)和分子量等物理化学特性差异较大, 我们初步选择了MeOH、ACN、EA和DCM作为超声萃取溶剂进行了基质加标回收试验, 如图 3所示。采用硅藻土作为空白基质, 使用前硅藻土在马弗炉中以460 ℃灼烧6 h去除其中干扰杂质。
![]() |
图 3 不同极性超声提取溶剂下OPEs的加标回收率 Fig. 3 Recoveries of organophosphate esters (OPEs) spiked in blank matrix with different polar extraction solvents |
在强极性溶剂MeOH提取物中, 只有TMP、TCPP、磷酸三丁酯(TBP)、磷酸三正戊酯(TPeP)和TEHP回收率较高, 其他OPEs的回收率都低于75%。EA、DCM和ACN提取物中, 多数OPEs的回收率较高在80%~125%之间, 但DCM对动物和人类具有致癌性(Watanabe et al, 2006), 世界卫生组织国际癌症研究机构将其列为2A类致癌物。此外, Martínez-Carballo等(2007)测定了奥地利水生环境中的9种OPEs, 也报道了DCM不适合用于超声提取技术从沉积物样品中提取OPEs, 故不考虑作为超声提取溶剂。由于OPEs的logKow值从–0.65到9.49, 大多数为正值, 说明OPEs的整体上亲脂性大于亲水性, 考虑对EA和ACN两种中强极性有机溶剂按不同体积比混合, 进一步优化提取效率。
EA和ACN对较亲脂的OPEs回收率相近, 但EA的极性为4.3, 对亲水性和极性较强的TMP、TEP、磷酸三异丙酯(TiPP)和磷酸三丙酯(TPrP)等回收率略低于极性为6.2的ACN, 故以EA作为少量组分, ACN作为大量组分进行混合配比。分别考察EA和ACN体积配比为2︰8、4︰6和3︰7三种混合溶剂对OPEs的提取效率。结果如图 4所示, 对亲脂性OPEs, EA/CAN (体积比3︰7)混合溶剂的回收率较高。对亲水性OPEs, EA/CAN (体积比3︰7)混合溶剂对卤代OPEs中TCEP (logKow=1.44)、∑TCPP (logKow=2.59)和V6 (logKow=1.9)的提取效果较有优势, 且回收率明显高于其他两种体积配比, 对TMP (logKow= –0.65)、TEP (logKow=0.8)、TPrP (logKow=1.87)和TiPP (logKow=2.12)四种非卤代OPEs的提取效果相对差一些。此外, EA/ACN(体积比3︰7)混合溶剂虽相较于其他两种体积配比条件下质谱图响应略低, 但基线平稳, 出峰更完整, 峰形更好, 如图 5所示。
![]() |
图 4 EA和ACN不同混合比条件下OPEs的加标回收率 Fig. 4 Recoveries of organophosphate esters (OPEs) spiked in blank matrix at different mixing ratios of ethyl acetate (EA) and acetonitrile (ACN) |
![]() |
图 5 EA/CAN (体积比4︰6)(a), EA/CAN (体积比3︰7)(b), EA/CAN (体积比2︰8)(c)混合溶剂萃取OPEs的色谱图对比 Fig. 5 Comparison of chromatograms of organophosphate esters (OPEs) extracted by ethyl acetate (EA) / acetonitrile (CAN) (4︰6, V︰V) (a), EA/CAN (3︰7, V︰V) (b), and EA/CAN (2︰8, V︰V) (c) mixed solvents at different volume ratios |
对比图 3和图 4, 单一有机溶剂EA的提取回收率范围为10%~125%, 平均回收率为93.28%, ACN的提取回收率范围为10%~120%, 平均回收率为90.15%。EA/ACN(体积比3︰7)混合溶剂的提取回收率范围为20%~140%, 平均回收率为105.98%, 因此选择EA/ACN(体积比3︰7)混合溶剂作为超声提取溶剂。
分别对20、30和40 mL三种超声溶剂体积的提取效果进行了对比, 如图 6所示。发现用30和40 mL超声溶剂体积提取时OPEs的回收率整体相近, 又有TMP、TPrP、TiPP三种极性较强的亲水性OPEs在40 mL超声体积提取时回收率极低, 而30 mL超声体积提取时回收率达到最佳。考虑到处理效果和节约溶剂两方面, 故选择使用30 mL超声体积。
![]() |
图 6 不同超声体积下OPEs的加标回收率 Fig. 6 Recoveries of organophosphate esters (OPEs) spiked in blank matrix of different volumes of solvent in ultrasonic extraction |
本文在中性条件下对CNWBOND LC-C18 SPE (6 mL/500 mg)、CNWBOND Si硅胶SPE (6 mL/500 mg)和Oasis®HLB 6 cc/500 mg三种SPE柱进行比较, 如图 7所示。Si硅胶柱的回收率明显是最差的, 故不考虑。LC-C18柱和HLB柱对强极性和强亲水性化合物的回收率都较差, 特别是易挥发性化合物TMP (logKow= –0.65), 萃取时易挥发损失导致回收率降低(Quintana et al, 2008, Reemtsma et al, 2008), HLB柱为2.82%, LC-C18柱为2.01%。但LC-C18柱对较亲水性OPEs(TEP、TCEP、TPrP、TiPP和TCPP)的洗脱效果优于HLB柱。疏水性化合物易在瓶壁吸附损失而使回收率降低, LC-C18柱对分子量大于600的疏水性OPEs [双酚A双(磷酸二苯酯)(BADP)、三(2, 3-二溴丙基)磷酸酯(TDBPP)和三(三溴新戊基)磷酸酯(TTBNP)等]洗脱效果差。HLB柱对强疏水性和非挥发性化合物TEHP (logKow=9.49)的回收率差(高立红等, 2014; 闫振飞等, 2020), 而LC-C18柱对TEHP的回收率优于HLB柱, HLB柱为8.86%, LC-C18柱为45.06%。
![]() |
图 7 不同SPE柱下OPEs的加标回收率 Fig. 7 Spiked recoveries of organophosphate esters (OPEs) in different solid phase extraction cartridges |
总体而言LC-C18固相萃取柱洗脱效果最好, 回收率在40%~150%之间, 平均回收率达到98.58%; HLB固相萃取柱次之, 回收率在20%~135%之间, 平均回收率为89.73%; 硅胶柱洗脱效果最差, 回收率在10%~100%之间, 平均回收率为38%。Been等(2018)基于回收率和基质效应, 评价了不同SPE的吸附剂及其提取条件, 得出C18柱对OPEs的处理效果最好, 与本研究结果一致。综合考虑选择LC-C18柱进行洗脱纯化。
进一步优化洗脱体积, 分别用5、6和7 mL的EA过LC-C18柱洗脱2次, 如图 8所示。发现5 mL洗脱体积对目标化合物的回收率略优, 但无特别显著的差异, 说明洗脱时间和挥发作用对LC-C18柱的洗脱效果影响不大。同时秉持绿色节约原则, 尽量节省试剂用量, 综上选取5 mL EA作为SPE的洗脱体积。
![]() |
图 8 不同洗脱体积下OPEs的加标回收率 Fig. 8 Spiked recoveries of organophosphate esters (OPEs) in different elution volumes |
配制一系列浓度为5、10、20、50、100、200和500 ng/mL的七点标准曲线, 并进行多次仪器分析, 在(5~500) ng/mL线性范围内各OPEs呈现良好的线性关系, 线性相关系数(R2)均达到0.99以上。
方法检出限(method detection limit, MDL)受仪器的灵敏度、稳定性、全程序空白试验以及波动性的影响。先确定仪器检出限(3倍信噪比), 然后重复7次空白样加标实验, 加标量是3倍仪器检出限对应浓度, 再以下式计算(白政忠等, 2009; 祝旭初, 2014):
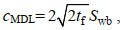
式中, cMDL为检出浓度; tf为0.05(单侧)显著性水平下, 自由度为f=n–1的t值; Swb为空白批内样本标准偏差。
定量限(limits of quantification, LOQ)定义为l0倍空白标准偏差对应的浓度值, 置信水平为90%, 约为3.3倍MDL (丁怡等, 2009; 陈爽等, 2014)。该方法测定27种OPEs的MDL的范围为0.004 ~1.250 ng/g之间, LOQ范围为0.01~4.17 ng/g, 见表 3。
化合物 | 线性范围/(ng/mL) | R2 | 检出限浓度/(ng/g) | 定量限浓度/(ng/g) |
TMP | 5~500 | 0.998 6 | 0.039 | 0.13 |
TEP | 5~500 | 0.999 5 | 0.039 | 0.13 |
TCEP | 5~500 | 0.998 7 | 0.636 | 2.12 |
TPrP | 5~500 | 0.999 4 | 0.006 | 0.02 |
TiPP | 5~500 | 0.998 5 | 0.014 | 0.05 |
V6 | 5~500 | 0.999 7 | 0.090 | 0.30 |
TPP | 5~500 | 0.998 6 | 0.039 | 0.13 |
TCPP | 5~500 | 0.997 4 | 0.039 | 0.20 |
(1, 2-)TCPP | 5~500 | 0.997 6 | 0.172 | 0.57 |
2-TCPP | 5~500 | 0.997 5 | 0.209 | 0.70 |
B(2, 3-d)P | 5~500 | 0.999 3 | 0.004 | 0.01 |
CDP | 5~500 | 0.999 4 | 0.023 | 0.08 |
TBP | 5 ~ 500 | 0.997 9 | 0.355 | 1.18 |
mono & di | 5 ~ 500 | 0.992 9 | 1.25 | 4.17 |
TBEP | 5~500 | 0.999 2 | 0.039 | 0.13 |
RDP | 5~500 | 0.999 5 | 0.013 | 0.04 |
TOCP | 5~500 | 0.997 7 | 0.116 | 0.39 |
TPCP | 5~500 | 0.998 3 | 0.099 | 0.33 |
TMTP | 5~500 | 0.997 6 | 0.088 | 0.29 |
EHDPP | 5~500 | 0.998 5 | 0.039 | 0.13 |
TPeP | 5~500 | 0.998 4 | 0.073 | 0.24 |
BADP | 5~500 | 0.999 2 | 0.234 | 0.78 |
TDBPP | 5~500 | 0.999 2 | 0.028 | 0.09 |
TiPPP | 5~500 | 0.999 1 | 0.096 | 0.32 |
TTBNP | 5~500 | 0.997 1 | 0.157 | 0.52 |
DPDP | 5~500 | 0.999 3 | 0.039 | 0.13 |
TEHP | 5~500 | 0.996 9 | 0.069 | 0.23 |
采用硅藻土作为基质来评价方法的准确度和精密度, 用最佳方案做基质加标实验。在空白基质中分别加入OPEs混合标准, 最终硅藻土中OPEs的含量分别为5、35和100 ng/g, 每个浓度设置3个基质加标平行样品。三个加标水平下各OPEs的平均加标回收率在45%~130%, 除TTBNP在低浓度和中等浓度加标水平下分别为25.43%和30.37%, 高浓度加标水平下TMP、BADP和TDBPP分别为37.55%、44.69%和43.01%。相对标准偏差(relative standard deviations, RSD)在0.03%~11.00%之间, 满足准确度和精密度要求, 如表 4所示。
化合物 | 加标水平/(ng/g) | |||||
5 | 35 | 100 | ||||
平均 回收率/% |
RSD/% | 平均回收率/% | RSD/% | 平均回收率/% | RSD/% | |
TMP | 45.12 | 1.02 | 57.10 | 1.79 | 37.55 | 0.03 |
TEP | 123.6 | 3.06 | 109.4 | 6.31 | 115.2 | 4.35 |
TCEP | 126.1 | 3.06 | 117.9 | 6.31 | 112.8 | 4.35 |
TPrP | 119.8 | 3.14 | 114.1 | 3.45 | 103.9 | 5.38 |
TiPP | 72.31 | 4.66 | 102.4 | 7.64 | 113.1 | 7.60 |
TCPP | 117.1 | 7.08 | 112.0 | 8.25 | 97.08 | 1.35 |
V6 | 107.7 | 1.77 | 101.5 | 3.72 | 104.5 | 10.6 |
TPP | 129.1 | 4.47 | 121.0 | 2.54 | 118.5 | 1.21 |
(1, 2-)TCPP | 119.0 | 5.52 | 113.9 | 6.55 | 109.8 | 8.79 |
2-TCPP | 121.6 | 5.07 | 115.5 | 8.03 | 104.0 | 8.67 |
B(2, 3-d)P | 89.08 | 5.07 | 84.51 | 9.56 | 88.30 | 5.67 |
CDP | 128.3 | 4.95 | 113.2 | 6.87 | 115.2 | 11.0 |
TBP | 87.91 | 1.31 | 94.44 | 9.47 | 86.18 | 7.37 |
TBEP | 104.0 | 7.92 | 96.30 | 3.73 | 96.79 | 6.68 |
RDP | 92.26 | 7.36 | 82.15 | 8.32 | 80.61 | 4.18 |
TMTP | 116.4 | 1.49 | 105.2 | 2.39 | 103.9 | 5.54 |
TOCP | 115.4 | 5.53 | 103.8 | 10.7 | 103.7 | 5.79 |
TPCP | 117.2 | 1.15 | 103.0 | 2.67 | 103.5 | 6.20 |
EHDPP | 103.9 | 8.84 | 92.28 | 9.16 | 88.41 | 6.22 |
TPeP | 105.3 | 2.10 | 98.43 | 1.34 | 94.73 | 10.9 |
BADP | 51.64 | 7.69 | 51.10 | 0.48 | 44.69 | 1.72 |
TDBPP | 55.15 | 9.76 | 51.27 | 5.51 | 43.01 | 2.06 |
DPDP | 86.07 | 2.83 | 78.91 | 3.21 | 71.41 | 5.77 |
TiPPP | 60.42 | 8.18 | 57.55 | 1.09 | 52.45 | 9.55 |
TTBNP | 25.43 | 9.74 | 30.37 | 6.34 | 75.28 | 3.45 |
Mono & di | 120.1 | 7.67 | 106.7 | 8.06 | 55.53 | 3.30 |
TEHP | 48.46 | 1.94 | 52.47 | 5.68 | 50.96 | 4.84 |
注: RSD表示相对标准偏差 |
采用建立的分析方法对东黄海域16个沉积物样品中27种OPEs进行测定, 样品站位点如图 9所示。27种OPEs中只有V6、TBP、磷酸三间甲苯酯(TMTP)、三邻甲苯基磷酸酯(TOCP)、三对甲苯基磷酸酯(TPCP)和TPeP没有检出, 检出的21种OPEs浓度范围为9.83 ng/g (站位CJ-4)~48.7 ng/g (站位3100-3), 平均浓度为28.4 ng/g, 各站点OPEs的检测浓度值如表 5所示。
![]() |
图 9 东黄海沉积物采样站位图 Fig. 9 Distribution of sediment sampling stations in the Yellow Sea and East China Sea |
检出化合物 | Za-1 | Za-2 | Za-3 | Za-4 | 3100-1 | 3100-2 | 3100-3 | 3100-4 | 3100-5 | 3100-6 | CJ-1 | CJ-2 | CJ-3 | CJ-4 | CJ-5 | CJ-6 |
TMP | 0.039 | 0.070 | 0.074 | ND | ND | ND | 0.050 | 0.045 | ND | ND | ND | ND | ND | ND | ND | ND |
TEP | 12.400 | 15.800 | 17.800 | 19.800 | 17.500 | 22.700 | 30.100 | 17.300 | 21.600 | 21.300 | 3.030 | 0.050 | 0.067 | 0.067 | 0.042 | 0.049 |
TCEP | 1.520 | 0.880 | 2.100 | 1.430 | 1.410 | 0.993 | 1.400 | 1.280 | 1.110 | 1.280 | 1.070 | 1.100 | 0.789 | ND | 1.280 | 1.610 |
TPrP | 0.036 | 0.047 | 0.021 | 0.017 | ND | 0.011 | 0.009 | 0.010 | 0.013 | 0.010 | 0.011 | ND | 0.006 | ND | 0.008 | 0.045 |
TiPP | 0.036 | 0.047 | 0.021 | ND | ND | ND | ND | ND | ND | ND | ND | ND | ND | ND | ND | 0.045 |
Mono & di | ND | ND | ND | 2.690 | ND | ND | 2.540 | 2.660 | ND | 2.480 | ND | 4.360 | ND | ND | ND | ND |
B(2, 3-d)P | 0.015 | 0.005 | ND | 0.014 | 0.007 | 0.008 | 0.008 | 0.005 | 0.013 | ND | ND | ND | ND | 0.012 | 0.006 | ND |
CDP | 0.126 | 0.033 | 0.048 | 0.087 | 0.123 | 0.088 | 0.088 | 0.110 | 0.088 | 0.088 | 0.069 | 0.128 | 0.066 | 0.026 | 0.062 | 0.067 |
TBEP | 0.125 | 0.065 | 0.091 | 0.110 | 0.091 | 0.048 | ND | 0.050 | ND | ND | 0.120 | 0.040 | ND | ND | 0.047 | ND |
RDP | 0.081 | ND | 0.022 | 0.033 | ND | 0.069 | ND | ND | 0.062 | 0.071 | ND | ND | ND | 0.018 | 0.027 | ND |
TEHP | 1.520 | 2.190 | 3.010 | 0.969 | 0.689 | 0.553 | 0.329 | 0.277 | 4.820 | 0.630 | 0.401 | 0.948 | 0.142 | 0.230 | 0.182 | 1.490 |
EHDPP | 0.124 | 0.124 | 0.145 | 0.153 | 0.046 | 0.154 | 0.072 | 0.055 | 0.108 | 0.050 | 0.055 | 0.150 | 0.055 | 0.084 | 0.077 | 0.047 |
TCPP | 4.420 | 2.380 | 3.420 | 3.280 | 3.610 | 2.270 | 5.870 | 4.980 | 3.330 | 5.180 | 4.37 | 3.250 | 2.100 | 0.541 | 6.370 | 6.030 |
(1, 2-) TCPP | 2.620 | 2.030 | 1.930 | 2.740 | 2.250 | 2.730 | 2.630 | 2.770 | 2.760 | 2.620 | 2.54 | 2.570 | 2.620 | 2.570 | 2.740 | 1.890 |
2-TCPP | 2.610 | 2.040 | 1.920 | 2.700 | 2.230 | 2.720 | 2.590 | 2.740 | 2.730 | 2.610 | 2.54 | 2.570 | 2.610 | 2.570 | 2.730 | 1.900 |
TPP | 2.650 | 2.030 | 1.950 | 2.750 | 2.270 | 2.720 | 2.630 | 2.810 | 2.680 | 2.630 | 2.590 | 2.590 | 2.650 | 2.590 | 2.730 | 1.880 |
BADP | ND | ND | ND | ND | ND | 0.691 | ND | ND | 0.694 | 0.405 | ND | 0.493 | ND | ND | ND | ND |
TTBNP | ND | ND | ND | ND | ND | 0.421 | ND | ND | 0.257 | 0.186 | ND | ND | ND | ND | ND | ND |
TiPPP | ND | ND | ND | ND | ND | 0.449 | ND | ND | 0.304 | 0.203 | ND | ND | ND | ND | ND | ND |
TDBPP | 0.151 | 0.131 | 0.100 | ND | 0.084 | 0.676 | 0.117 | 0.105 | 0.720 | 0.413 | 0.208 | 0.454 | ND | 0.046 | 0.063 | ND |
DPDP | 0.056 | ND | ND | ND | ND | 0.137 | ND | ND | 0.077 | 0.079 | ND | ND | ND | ND | ND | ND |
注: ND表示未检出 |
16个沉积物样品中的OPEs包括烷基代、卤代和芳基代三种取代基类型, 检出的21种OPEs中烷基代、卤代和芳基代各7种, 其中TEP、磷酸三苯酯(TPP)和∑TCPP为主要化合物且检出率均为100%。烷基代OPEs对OPEs总浓度(∑OPEs)的贡献率为52.85%, 高于卤代OPEs(贡献率为36.46%)和芳基代OPEs仅为10.68%, 如图 10a所示。从图 10b、10c、10d和表 5中得出, 东、黄海交界处(如CJ-样品) ∑TCPP和TPP浓度明显高于其他站位点, 可能由于我国最大的OPEs生产地之一位于黄海南部的西海岸, 年产量2万t, 主要产物为TCPP和TDCPP (Zhong et al, 2018), 且江苏省南部海岸有冶金、电子、化学和机械等多个行业(Zhong et al, 2017), 而TPP在电子设备中用作阻燃剂(Andresen et al, 2006)。TEP在石油化工副产品PVC中用作增塑剂(Van der Veen et al, 2012), 故位于长江三角洲的石油化工项目集中区废液排放导致TEP在东海中的含量也很高(如Za-样品和3100-样品), 另外长江入海口输入以及杭州湾与东海海水的混合交换也可能是TEP等的潜在来源。这意味着东海检出的OPEs可能是河流输入和排污口排放所致, 与Zhong等(2018)对渤海、黄海和东海表层沉积物中OPEs的研究及Liao等(2020)对渤海和东海沉积物表层、岩芯中OPEs的研究结果一致。
![]() |
图 10 沉积物中OPEs的组成特征(a)和烷基代OPEs(b), 卤代OPEs(c), 芳基代OPEs(d)浓度百分比组成 Fig. 10 Composition profiles of organophosphate esters (OPEs) in the sediments (a) and the percentage composition of alkylated OPEs concentrations (b), halogenated OPEs concentrations (c), arylated OPEs concentrations (d) |
(1) 本研究建立了同时测定海洋沉积物中27种OPEs的方法。改进HPLC-MS/MS液相条件, 流动相为甲醇和含0.002 5%的甲酸水溶液时, 大大提高了MRM质谱扫描模式分析目标化合物的仪器响应。又对沉积物前处理的超声提取和固相萃取条件加以优化, 30 mL ACN/EA (体积比3︰7)超声提取, 处理液用5 mL EA经LC-C18固相萃取小柱洗脱, 回收率高且节约了溶剂用量。优化后方法的MDL和LOQ满足沉积物中痕量污染物可检出和定量的要求, 低、中、高加标水平下各OPEs的平均加标回收率较高, 平行性较好。该方法高效灵敏, 可进一步推进到海水OPEs检测方法的建立中, 为测定海洋水体环境中痕量OPEs提供可靠的技术支撑。
(2) 利用建立好的方法对东黄海16个沉积物样本进行了分析测定, 检出的21种OPEs浓度范围为9.83~48.7 ng/g, 平均浓度为28.4 ng/g, 其中烷基代OPEs占比最高(52.85%)。发现东黄海沉积物中OPEs主要来源于河流输入和沿岸的点源污染排放, 需要进一步加强对东黄海OPEs的分布特征和迁移转化规律方面的研究, 为海洋环境中持久性痕量污染物的控制以及生态风险评估提供有力依据。
丁怡, 彭程, 2009. 多种方法评估液相色谱方法检出限的初步探讨. 现代食品科技, 25(11): 1372-1374, 1314 |
王九明, 陈军辉, 杨建勃, 等, 2021. 固相萃取-液相色谱-串联质谱法测定海水中软骨藻酸. 色谱, 39(8): 889-895 |
邓旭, 印红玲, 何婉玲, 等, 2019. 有机磷酸酯在成都市市/郊区剖面土壤及农作物中的分布及迁移. 环境化学, 38(3): 679-685 |
白政忠, 张秋生, 2009. 应关注低浓度药物分析方法检出限(MDL)的确定. 中国药品标准, 10(6): 405-407 DOI:10.3969/j.issn.1009-3656.2009.06.002 |
印红玲, 李世平, 叶芝祥, 等, 2016. 成都市土壤中有机磷阻燃剂的污染特征及来源分析. 环境科学学报, 36(2): 606-613 |
刘娴静, 梁存珍, 肖本益, 等, 2021. 高效液相色谱-串联质谱法同时检测水体中26种药物及个人护理品. 环境化学, 40(2): 549-558 |
闫振飞, 廖伟, 冯承莲, 等, 2020. 典型有机磷酸酯阻燃剂分析方法研究进展. 生态毒理学报, 15(1): 94-108 |
吴迪, 印红玲, 李世平, 等, 2019. 成都市锦江表层水和沉积物中有机磷酸酯的污染特征. 环境科学, 40(3): 1245-1251 DOI:10.3969/j.issn.1000-6923.2019.03.042 |
陈爽, 徐接胜, 2014. 关于检出限的定义、分类及估算方法的探讨. 广州化工, 42(18): 137-139 DOI:10.3969/j.issn.1001-9677.2014.18.052 |
祝旭初, 2014. 空白批内标准偏差和方法检出限的计算. 化学分析计量, 23(3): 96-98 DOI:10.3969/j.issn.1008-6145.2014.03.028 |
耿存珍, 段玉双, 王艺璇, 等, 2016. 有机磷系阻燃剂的全球污染现状. 生态毒理学报, 11(2): 124-133 |
高立红, 厉文辉, 史亚利, 等, 2014. 有机磷酸酯阻燃剂分析方法及其污染现状研究进展. 环境化学, 33(10): 1750-1761 DOI:10.7524/j.issn.0254-6108.2014.10.004 |
郭志明, 刘頔, 申铠君, 等, 2016. 太原市PM2.5中有机磷阻燃剂初步研究: 污染特征及季节变化. 地球与环境, 44(6): 600-604 |
ANDRESEN J, BESTER K, 2006. Elimination of organophosphate ester flame retardants and plasticizers in drinking water purification. Water Research, 40(3): 621-629 DOI:10.1016/j.watres.2005.11.022 |
BEEN F, BASTIAENSEN M, LAI F Y, et al, 2018. Mining the chemical information on urban wastewater: monitoring human exposure to phosphorus flame retardants and plasticizers. Environmental Science & Technology, 52(12): 6996-7005 |
BJÖRKLUND J, ISETUN S, NILSSON U, 2004. Selective determination of organophosphate flame retardants and plasticizers in indoor air by gas chromatography, positive-ion chemical ionization and collision-induced dissociation mass spectrometry. Rapid Communications in Mass Spectrometry, 18(24): 3079-3083 DOI:10.1002/rcm.1721 |
BOLLMANN U E, MÖLLER A, XIE Z Y, et al, 2012. Occurrence and fate of organophosphorus flame retardants and plasticizers in coastal and marine surface waters. Water Research, 46(2): 531-538 DOI:10.1016/j.watres.2011.11.028 |
CAMPO J, LORENZO M, CAMMERAAT E L H, et al, 2017. Emerging contaminants related to the occurrence of forest fires in the Spanish Mediterranean. Science of the Total Environment, 603-604: 330-339 DOI:10.1016/j.scitotenv.2017.06.005 |
CHEN Y Q, ZHANG Q, LUO T W, et al, 2019. Occurrence, distribution and health risk assessment of organophosphate esters in outdoor dust in Nanjing, China: urban vs. rural areas. Chemosphere, 231: 41-50 DOI:10.1016/j.chemosphere.2019.05.135 |
CHOI W, LEE S, LEE H K, et al, 2020. Organophosphate flame retardants and plasticizers in sediment and bivalves along the Korean coast: occurrence, geographical distribution, and a potential for bioaccumulation. Marine Pollution Bulletin, 156: 111275 DOI:10.1016/j.marpolbul.2020.111275 |
CRISTALE J, GARCÍA VÁZQUEZ A, BARATA C, et al, 2013. Priority and emerging flame retardants in rivers: Occurrence in water and sediment, Daphnia magna toxicity and risk assessment. Environment International, 59: 232-243 DOI:10.1016/j.envint.2013.06.011 |
KIM U J, KANNAN K, 2018. Occurrence and distribution of organophosphate flame retardants/plasticizers in surface waters, tap water, and rainwater: implications for human exposure. Environmental Science & Technology, 52(10): 5625-5633 |
LI J, YU N Y, ZHANG B B, et al, 2014. Occurrence of organophosphate flame retardants in drinking water from China. Water Research, 54: 53-61 DOI:10.1016/j.watres.2014.01.031 |
LIAO C Y, KIM U J, KANNAN K, 2020. Occurrence and distribution of organophosphate esters in sediment from northern Chinese coastal waters. Science of the Total Environment, 704: 135328 DOI:10.1016/j.scitotenv.2019.135328 |
LUO H Y, XIAN Y P, GUO X D, et al, 2014. Dispersive liquid-liquid microextraction combined with ultrahigh performance liquid chromatography/tandem mass spectrometry for determination of organophosphate esters in aqueous samples. The Scientific World Journal, 2014: 162465 |
MA Y X, XIE Z Y, LOHMANN R, et al, 2017. Organophosphate ester flame retardants and plasticizers in ocean sediments from the north pacific to the arctic ocean. Environmental Science & Technology, 51(7): 3809-3815 |
MARTÍNEZ-CARBALLO E, GONZÁLEZ-BARREIRO C, SITKA A, et al, 2007. Determination of selected organophosphate esters in the aquatic environment of Austria. Science of the Total Environment, 388(1/2/3): 290-299 |
NA G S, HOU C, LI R J, et al, 2020. Occurrence, distribution, air-seawater exchange and atmospheric deposition of organophosphate esters (OPEs) from the Northwestern Pacific to the Arctic Ocean. Marine Pollution Bulletin, 157: 111243 DOI:10.1016/j.marpolbul.2020.111243 |
PANTELAKI I, VOUTSA D, 2020. Occurrence, analysis and risk assessment of organophosphate esters (OPEs) in biota: a review. Marine Pollution Bulletin, 160: 111547 DOI:10.1016/j.marpolbul.2020.111547 |
PEVERLY A A, O'SULLIVAN C, LIU L Y, et al, 2015. Chicago's sanitary and ship canal sediment: polycyclic aromatic hydrocarbons, polychlorinated biphenyls, brominated flame retardants, and organophosphate esters. Chemosphere, 134: 380-386 DOI:10.1016/j.chemosphere.2015.04.065 |
QUINTANA J B, RODIL R, REEMTSMA T, et al, 2008. Organophosphorus flame retardants and plasticizers in water and air II. Analytical methodology. TrAC Trends in Analytical Chemistry, 27(10): 904-915 DOI:10.1016/j.trac.2008.08.004 |
RAUERT C, SCHUSTER J K, ENG A, et al, 2018. Global atmospheric concentrations of brominated and chlorinated flame retardants and organophosphate esters. Environmental Science & Technology, 52(5): 2777-2789 |
REEMTSMA T, QUINTANA J B, RODIL R, et al, 2008. Organophosphorus flame retardants and plasticizers in water and air I. Occurrence and fate. TrAC Trends in Analytical Chemistry, 27(9): 727-737 DOI:10.1016/j.trac.2008.07.002 |
REN G F, CHU X D, ZHANG J, et al, 2019. Organophosphate esters in the water, sediments, surface soils, and tree bark surrounding a manufacturing plant in North China. Environmental Pollution, 246: 374-380 DOI:10.1016/j.envpol.2018.12.020 |
RODIL R, QUINTANA J B, REEMTSMA T 2005. Liquid chromatography-tandem mass spectrometry determination of nonionic organophosphorus flame retardants and plasticizers in wastewater samples [J]. Analytical Chemistry, 77(10): 3083-3089.
|
SCHMIDT N, CASTRO-JIMÉNEZ J, OURSEL B, et al, 2021. Phthalates and organophosphate esters in surface water, sediments and zooplankton of the NW Mediterranean Sea: exploring links with microplastic abundance and accumulation in the marine food web. Environmental Pollution, 272: 115970 DOI:10.1016/j.envpol.2020.115970 |
SUNDKVIST A M, OLOFSSON U, HAGLUND P, et al, 2010. Organophosphorus flame retardants and plasticizers in marine and fresh water biota and in human milk. Journal of Environmental Monitoring, 12(4): 943-951 DOI:10.1039/b921910b |
SUO L L, HUANG W H, ZHU Q Y, et al, 2018. Accelerated solvent extraction coupled to high-performance liquid chromatography-tandem mass spectrometry for simultaneous determination of 11 organophosphorus flame retardants in aquatic products. Journal of the Science of Food and Agriculture, 98(14): 5287-5293 DOI:10.1002/jsfa.9067 |
TEO T L L, MCDONALD J A, COLEMAN H M, et al, 2015. Analysis of organophosphate flame retardants and plasticisers in water by isotope dilution gas chromatography- electron ionisation tandem mass spectrometry. Talanta, 143: 114-120 DOI:10.1016/j.talanta.2015.04.091 |
VAN DER VEEN I, DE BOER J, 2012. Phosphorus flame retardants: properties, production, environmental occurrence, toxicity and analysis. Chemosphere, 88(10): 1119-1153 DOI:10.1016/j.chemosphere.2012.03.067 |
WANG Y, WU X W, ZHANG Q N, et al, 2017. Organophosphate esters in sediment cores from coastal Laizhou Bay of the Bohai Sea, China. Science of the Total Environment, 607/608: 103-108 DOI:10.1016/j.scitotenv.2017.06.259 |
WANG X L, ZHONG W J, XIAO B W, et al, 2019. Bioavailability and biomagnification of organophosphate esters in the food web of Taihu Lake, China: impacts of chemical properties and metabolism. Environment International, 125: 25-32 DOI:10.1016/j.envint.2019.01.018 |
WANG X, ZHU Q Q, YAN X T, et al, 2020. A review of organophosphate flame retardants and plasticizers in the environment: analysis, occurrence and risk assessment. Science of the Total Environment, 731: 139071 DOI:10.1016/j.scitotenv.2020.139071 |
WATANABE K, GUENGERICH F P, 2006. Limited reactivity of formyl chloride with glutathione and relevance to metabolism and toxicity of dichloromethane. Chemical Research in Toxicology, 19(8): 1091-1096 DOI:10.1021/tx060087n |
WEI G L, LI D Q, ZHUO M N, et al, 2015. Organophosphorus flame retardants and plasticizers: Sources, occurrence, toxicity and human exposure. Environmental Pollution, 196: 29-46 DOI:10.1016/j.envpol.2014.09.012 |
WU Z J, LIU J P, PENG Y, 2017. QuEChERS purification prior to stable isotope dilution-ultra-high performance liquid chromatography tandem mass spectrometry to determine organophosphate esters of trace amounts in dairy products. Analytical Methods, 9(15): 2290-2298 DOI:10.1039/C6AY03162E |
XING L Q, ZHANG Q, SUN X, et al, 2018. Occurrence, distribution and risk assessment of organophosphate esters in surface water and sediment from a shallow freshwater Lake, China. Science of the Total Environment, 636: 632-640 DOI:10.1016/j.scitotenv.2018.04.320 |
ZENG X Y, XU L, HU Q P, et al, 2020. Occurrence and distribution of organophosphorus flame retardants/plasticizers in coastal sediments from the Taiwan Strait in China. Marine Pollution Bulletin, 151: 110843 DOI:10.1016/j.marpolbul.2019.110843 |
ZHONG M Y, TANG J H, MI L J, et al, 2017. Occurrence and spatial distribution of organophosphorus flame retardants and plasticizers in the Bohai and Yellow Seas, China. Marine Pollution Bulletin, 121(1/2): 331-338 |
ZHONG M Y, WU H F, MI W Y, et al, 2018. Occurrences and distribution characteristics of organophosphate ester flame retardants and plasticizers in the sediments of the Bohai and Yellow Seas, China. Science of the Total Environment, 615: 1305-1311 DOI:10.1016/j.scitotenv.2017.09.272 |
ZOU Y T, FANG Z, LI Y, et al, 2018. Novel method for in situ monitoring of organophosphorus flame retardants in waters. Analytical Chemistry, 90(16): 10016-10023 DOI:10.1021/acs.analchem.8b02480 |